The aim of our research is to understand how complex traits vary and evolve. Many traits of interest differ between individuals as a result of a complicated interplay between genetic differences, environmental differences, and random chance.
A major feature of many complex traits is their robustness (Masel & Siegal 2009). That is, the regulatory networks that produce them suppress the effects of both genetic and nongenetic perturbations. Our lab uses a variety of approaches to understand the causes and evolutionary consequences of this robustness. We also study cases where variability (or the lack of robustness) appears to be advantageous. Such cases include so-called bet-hedging mechanisms, whereby a population maximizes its long-term success in an uncertain environment by maintaining subpopulations that thrive under different conditions (Levy et al. 2012). Our studies of robustness have also led us to study genetic interactions that determine trait outcomes, and we have developed a powerful new approach for mapping loci that participate in such interactions (Buzby et al. 2024). Our work primarily focuses on the budding yeast, Saccharomyces cerevisiae, but has also involved another major model organism, the fly Drosophila melanogaster, as well as theoretical work involving computational simulations and analysis of human data from genome-wide association studies.
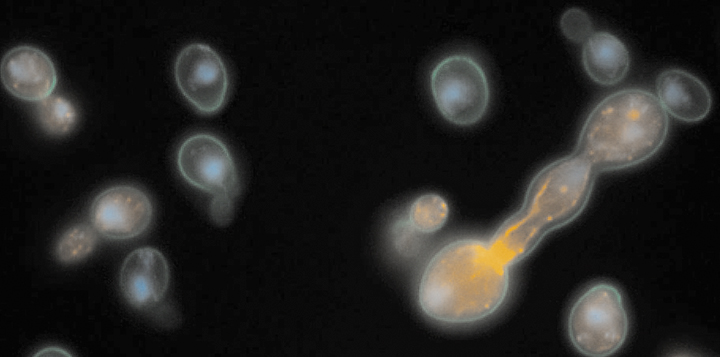
One major experimental focus in our lab is on directly identifying and characterizing genes that contribute to robustness of many traits. We have screened the genome of S. cerevisiae for genes whose deletion increases the variation in the morphologies of individual, genetically identical cells (i.e., the genes normally contribute to robustness against chance fluctuations in the external or internal cellular environment). Hundreds of nonessential yeast genes increase morphological variation when deleted, and these genes tend to be highly connected in cellular networks (Levy & Siegal 2008). An even greater proportion of essential genes contribute to robustness against environmental fluctuations (Bauer et al. 2015). We have also tested whether the same mechanisms that buffer environmental fluctuations also buffer the effects of mutations. A longstanding hypothesis in the field has been that the two types of buffering should be linked mechanistically, but we have refuted this connection in the case of the chromatin protein H2A.Z (Richardson et al. 2013) and the molecular chaperone Hsp90 (Geiler-Samerotte et al. 2016). Indeed, our work supports the view that selection against unbuffered alleles leaves the false impression that proteins such as Hsp90 preferentially buffer the effects of mutations (Geiler-Samerotte et al. 2016).
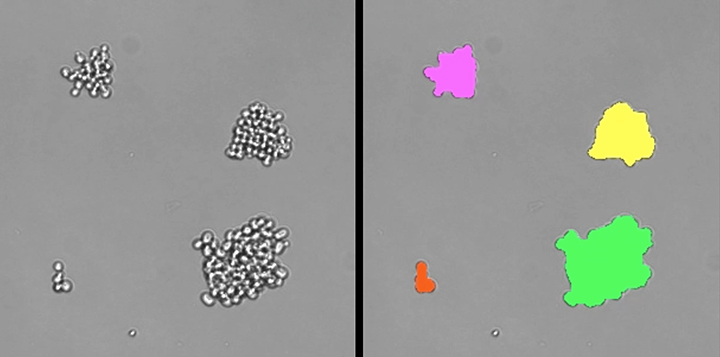
A second major experimental focus is on our discovery of a putative bet-hedging system in S. cerevisiae using a high-throughput, microscopy-based growth assay that we developed (Levy et al. 2012; Sartori et al. 2021). Individual yeast cells show a large amount of variation in growth rate, and these differences are transiently heritable. Slower-growing cells are naturally outcompeted by faster-growing cells when conditions are benign, but are better able to survive acute stress. Different strains of yeast differ in their growth-rate distributions, suggesting that ecological pressures might shape bet-hedging strategies in nature (Ziv et al. 2013; Ziv et al. 2017). We are currently working on trying to understand the molecular mechanism underlying heterogeneity in growth rate and stress resistance (Li et al. 2018), as well as its divergence between strains and species (Ziv et al. 2017).